Moisture Swing Adsorption for CO2 Capture
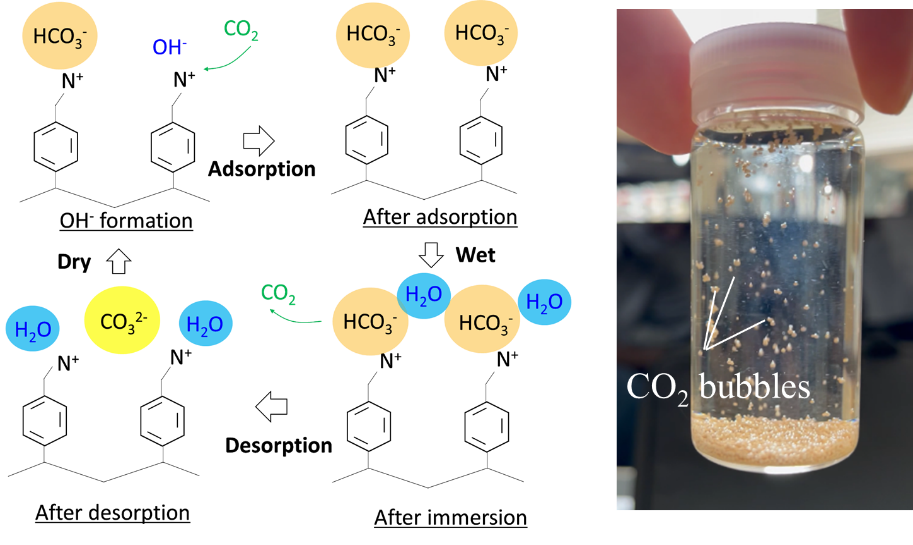
In recent years, efforts have been made to capture, store, and utilize CO2 from exhaust gases and the atmosphere. The key to this is CO2 capture technology. There are various methods such physical and chemical adsorption. However, the main challenge is to lower energy use. That’s why we are focusing on a method called moisture swing adsorption. In this method, CO2 is captured when the adsorbent is dry and released when it is wet. This process only requires water to release CO2, which could significantly reduce energy consumption compared to traditional CO2 capture methods that rely on heat.
(1) Development of adsorbent
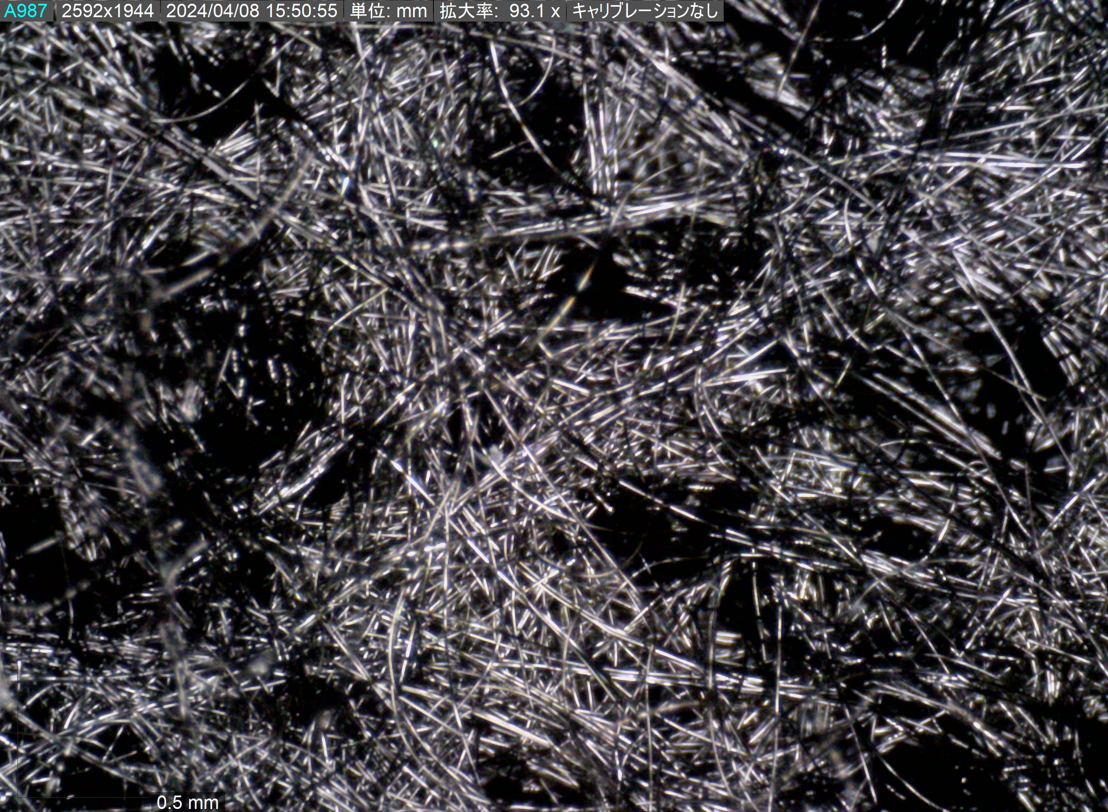
The CO2 adsorbent used in the moisture swing adsorption method needs to have both a high capacity and a fast adsorption rate for capturing CO2. Therefore, we are developing CO2 adsorbents with excellent performance by designing a porous material and the polymer structure.
(2) Elucidation of mass transport
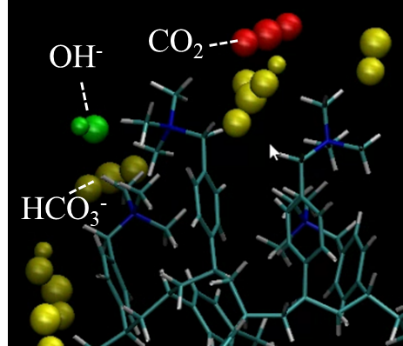
To make high-performance adsorbents, we need to understand how CO2 moves in polymers. Therefore, we are studying mass transport in polymers using molecular dynamics, which helps us suggest improved adsorbent structures. We are also working on a method to visualize CO2 inside these polymers.
(3) Energy management
Energy management of CO2 capture systems is crucial for lowering energy consumption. We have created a system that captures about 1.0 ton of CO2 per year from the atmosphere. Using this data, we are working to find the best setup and operating mode to minimize energy consumption in the CO2 capture system.
Organic synthesis by CO2 electrolysis
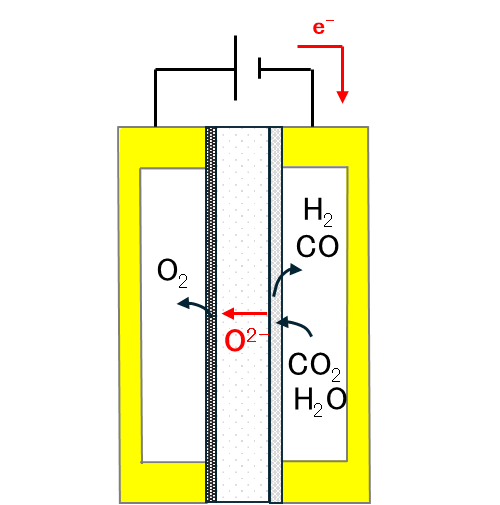
We are researching electrolysis technologies to convert CO2 captured by the moisture swing adsorption method into carbon monoxide (CO), which can be used to produce various organic materials. Specifically, we are examining how impurities in CO2 affect the electrolysis process, especially in polymer and solid oxide electrolysis. We are also working on creating more durable cell structures and systems. Additionally, we are developing cells that can effectively use the oxygen generated during CO2 electrolysis.
Turbulent jet ignition suitable for synthetic fuels
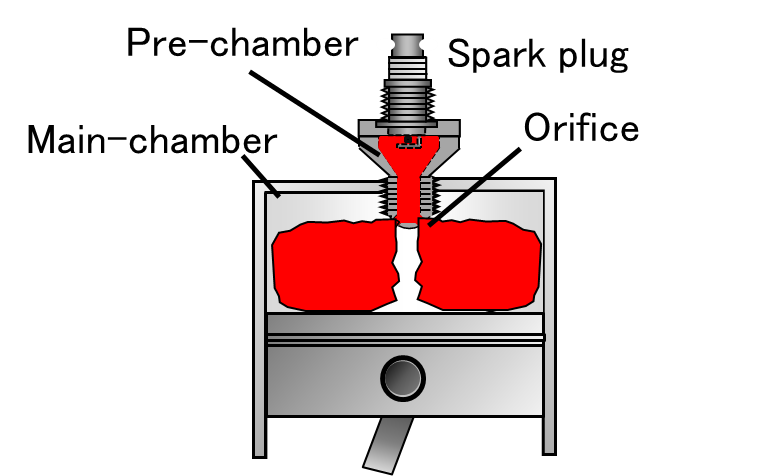
We are studying turbulent jet ignition, an efficient combustion technology that uses synthetic fuel made from CO2. In this method, fuel in the pre-chamber is ignited and burned, and the flame is then injected into the main chamber. This technology can shorten combustion duration and improve the engine's thermal efficiency. The shape of the orifice connecting the pre-chamber and main chamber is crucial for stable combustion. Therefore, we are investigating how turbulent jet ignition functions with synthetic fuels and optimizing the orifice shape using visualization experiments and numerical simulations that include chemical reactions. We are also working on developing synthetic fuels that can further improve thermal efficiency.
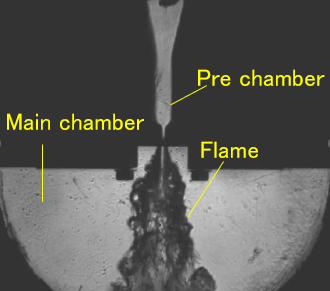
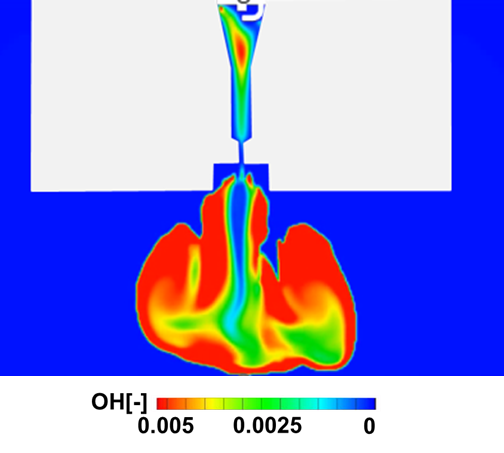
Polymer Electrolyte Fuel Cells
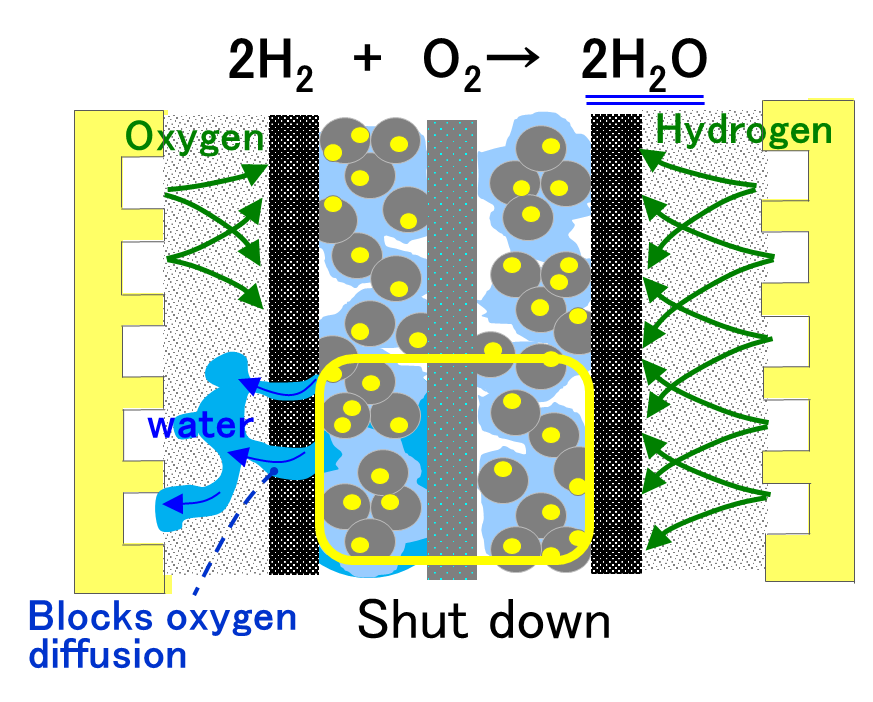
We are researching water and oxygen transport in polymer electrolyte fuel cells (PEFCs), which can efficiently use hydrogen as a decarbonization technology. In the PEFC, hydrogen and oxygen generate electricity, producing water as a byproduct. When this water accumulates in the cell, it blocks oxygen diffusion, so it needs to be properly managed. Therefore, we are working on designing cell structures that optimize the transport of water and oxygen.
(1) Two-phase flow simulation by lattice Boltzmann method
The PEFC contains microporous materials, making two-phase flow simulations very computationally intensive. To address this, we have developed a simulation method based on the lattice Boltzmann method. This method allows for stable solutions with large time steps and is well-suited for parallel calculations. We have also reduced the computational load by partially lowering the spatial resolution using a multi-block approach. Currently, we are working on a model that incorporates both water phase changes and oxygen transport. Our final goal is to develop a simulation model that can be used in the design stage of PEFC systems to optimize cell structures.
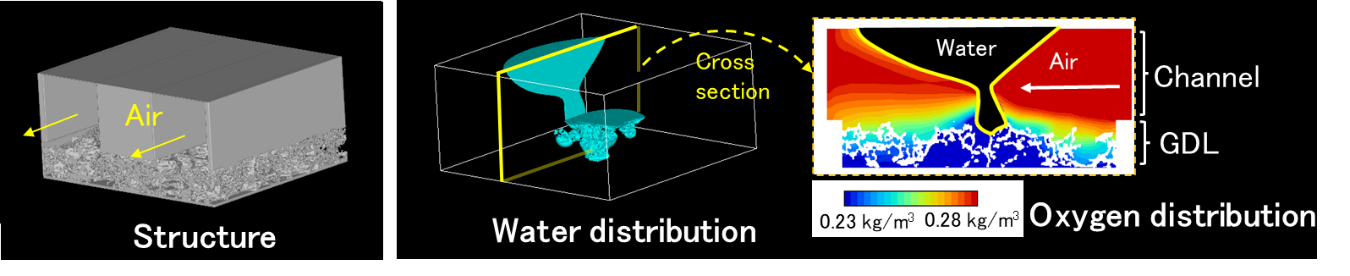
(2) Gas diffusion layer/microporous layer
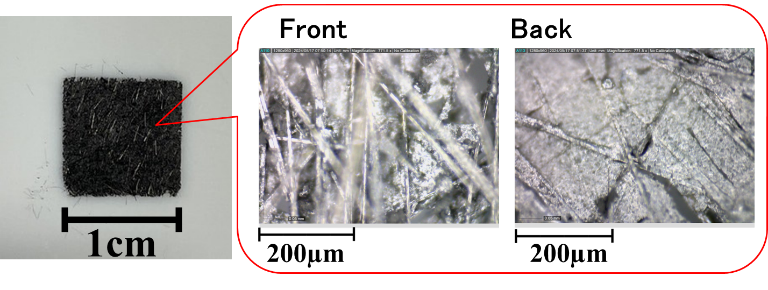
To enhance oxygen transport in PEFCs, it is effective to reduce the thickness of the gas diffusion layer and microporous layer. Therefore, we are developing these layers to be less than 40 µm thick. Additionally, we are working on technology to visualize the water content and temperature within the gas diffusion layers.